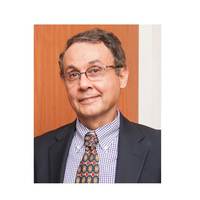
Dr Yehia F Khalil
1) Operating Agent for the International Energy Agency (IEA), Hydrogen Technologies Safety Program
2) Elected Fellow, University of Oxford, United Kingdom.
3) Elected member of Connecticut Academy of Science and Engineering (CASE)
4) Editor-in-chief, Hydrogen Safety Journal, International Energy Agency (IEA)
5) Editor, Process Safety and Environmental Protection (PSEP), Elsevier Journal, IChemE Publication, UK
6) Senior Editor, J. of Environmental Engineering &
Ecological Science, UK
7) Past Technical Fellow, Center for Advanced Engineering Studies, MIT, Cambridge, MA, USA
8) Past AIChE/Yale Faculty Advisor, Yale University, CT, USA
At Yale University, Prof. Khalil taught core courses in the chemical engineering curricula including Chemical Engineering Process Design (the capstone course in chemical engineering), Chemical Engineering Process Control, Separation & Purification, and the Chemical Engineering teaching Laboratory. He also taught Air Pollution Control (a core course in environmental engineering), Environmental Risk Assessment (ERA), Green Energy Systems (GES), and Environmental Control Technologies. Prof. Khalil advised undergraduate students in their senior projects and graduate students in their Master thesis.
2) Elected Fellow, University of Oxford, United Kingdom.
3) Elected member of Connecticut Academy of Science and Engineering (CASE)
4) Editor-in-chief, Hydrogen Safety Journal, International Energy Agency (IEA)
5) Editor, Process Safety and Environmental Protection (PSEP), Elsevier Journal, IChemE Publication, UK
6) Senior Editor, J. of Environmental Engineering &
Ecological Science, UK
7) Past Technical Fellow, Center for Advanced Engineering Studies, MIT, Cambridge, MA, USA
8) Past AIChE/Yale Faculty Advisor, Yale University, CT, USA
At Yale University, Prof. Khalil taught core courses in the chemical engineering curricula including Chemical Engineering Process Design (the capstone course in chemical engineering), Chemical Engineering Process Control, Separation & Purification, and the Chemical Engineering teaching Laboratory. He also taught Air Pollution Control (a core course in environmental engineering), Environmental Risk Assessment (ERA), Green Energy Systems (GES), and Environmental Control Technologies. Prof. Khalil advised undergraduate students in their senior projects and graduate students in their Master thesis.
less
InterestsView All (7)
Uploads
Papers by Dr Yehia F Khalil
Link:
https://www.ieahydrogen.org/tasks-reports/#17-17-task-reports-p2
The importance of integrating E-LCA and S-LCA for the deployment of hydrogen technologies is discussed, with an emphasis on the challenges of conducting S-LCA for low Technology Readiness Level (TRL) hydrogen production pathways. The study concludes that combining E-LCA and S-LCA results for product systems and processes can significantly contribute to the United Nations' seventeen Sustainable Development Goals (SDGs) and align with the UN's Environmental, Social, and Governance (ESG) dimensions. Incorporating this combined framework into ESG reporting enhances the accuracy, transparency, and credibility of sustainability disclosures, thereby supporting improved decision-making and stakeholder engagement. Key recommendations from the research include: a) the necessity of integrating E-LCA with S-LCA to provide comprehensive sustainability assessments and to identify environmental and social hotspots across hydrogen production value chains, and b) recognizing that sustainable hydrogen deployment must be driven by environmental and economic benefits, as well as social responsibility, worker well-being, labor rights, and occupational health and safety, among other important social indicators.
Keywords: Environmental hotspots; social hotspots; E-LCA; S-LCA; SHDB; PSILCA
Note: Interested readers can find more details in Khalil’s (1997) doctoral dissertation in management science (Sc.D.).
Unsurprisingly, the expected electricity price wars, battles for survival, and tremendous pressure exerted by big customers for rate reliefs are driving the electric utilities to sign bilateral agreements and energy deals with their major wholesale customers (commercial and industrial) in order to maintain the utility's market share and main sources of revenue. For example, Michigan investor-owned utility (IOU) company has lowered the electricity rates for its 9,000 industrial customers by 4.2% and a handful of large companies such as General Motors
(GM) have already received 10-20% rate cuts. However, the inevitable by-product of employing
this business practice is about 8.2% rates rise for Michigan 1.4 million captive customers
(residential and small business customers) to make up for the lost revenue from large
electricity customers. Furthermore, state regulators (viz., Department of Public Utility Commission ‘DPUC’) realize the benefits of maintaining in-state economic vitality and recognize utilities' fear of losing major revenues and the threat of the so-called "stranded investments" for utilities with nuclear assets. In response, unsurprisingly, regulators at the state level (viz., DPUC’) tend to bless these special electric rate contracts. While such deals provide the customers with discounted electricity rates, it ensures that the customer would buy most of its electricity needs from the utility rather than self-generating (for example, building its own gas-fired generator that supplies electricity and steam) or buying cheaper electricity from other competitors such as the Independent Power Producers (IPPs) and out-of-state utilities (under the so-called electricity retail wheeling schemes and transactions).
In this research, a hypothetical scenario of the bilateral agreements between an electric
utility and a wholesale price-sensitive customer is discussed. This scenario is simulated using Powersim system dynamics (SD) software to model this scenario development with complex dynamics using causal feedback loops, accumulation of flows into stocks and time delays. Also discussed are the short-term gains and unintended long-term consequences of potential strategic options such as offering premium / discount periods and lowering electricity production costs to make up for the price differentials.
Yehia F. Khalil1 and Tom Elicson2
Abstract
The authors of this research used the Modular Accident Analysis Programs MAAP 4.0.2 and MAAP4-DOSE to calculate the revised source term for regulatory applications in three parts as follows: 1) Source term release to containment, which reflects the likelihood of its occurrence, 2) Fission product distribution and removal in containment, and 3) Source term release to the environment including off-site dose calculations.
All three of these calculations were performed in a single, integrated analysis with MAAP 4 for the dominant core-melt sequences identified in the Millstone IPE.* Such calculations have numerous regulatory applications, including the relaxation or allowable leakage limits, the relaxation of primary and secondary containment isolation requirements, and revisions to surveillance specifications for safety-related equipment.
Reference
*Khalil, Y. F. (1992). Millstone Unit 1 Individual Plant Examination (IPE) for Severe accident Vulnerabilities. Northeast Utilities, NUSCO Report # 174, 1992.
____________
1 Engineering Manager, Probabilistic Risk Assessment, Northeast Utilities, Berlin, CT
Dr. Yehia F. Khalil is the corresponding author. [email protected]
2 Fauske & Associates (FAI), IL.
Y. F. Khalil, Ph.D., Sc.D.
Engineering Manager, Probabilistic Risk Assessment (PRA)
Millstone Nuclear Power Station, Waterford, CT, USA
Abstract
The U.S. Nuclear Regulatory Commission’s (NRC) generic letter GL 88-20 on ‘Severe Accident Vulnerabilities’ required the U.S. nuclear licensees with BWR and Mark-I containment units to assess potential containment failure mechanisms including drywell liner failure by melt-through.
The interest in this phenomenology surfaced after several research efforts at U.S. national laboratories (BNL and ORNL) predicted that ex-vessel molten corium attack on drywell liner could be a dominant failure mode and, therefore, would be a major concern to public safety.
The author of this theoretical study performed deterministic (using MAAP severe accident simulation program) and probabilistic analyses (using fault tree and event tree analyses) to assess the vulnerability of Millstone Unit 1 to drywell liner to melt-through and creep-rupture should it come in direct contact with molten corium. Accident management strategies were recommended to reduce drywell liner vulnerability to melt-through, mitigate the severity of radionuclide releases (given a liner melt-through), and reduce the likelihood of combustible gas burns in the auxiliary building, given a liner melt-though. Finally, potential accident management concerns were identified.
The insights gained from the Individual Plants Examinations (IPEs) for MP-1 and EF-2 are also discussed in this work. For the most part, this comparative theoretical study is confined to the Back-End, viz., Level-II Probabilistic Risk Assessment (PRA), analysis of the IPE.
References:
Khalil, Y.F. (1992). Millstone Unit 1 Individual Plant Examination for Severe Accident Vulnerabilities, Northeast Utilities, NUSCO 174, March 1992.
Solid-state hydrogen storage materials are a high-energy density option for a variety of hydrogen fuel cell applications including forklifts, automobiles, and portable battery replacement systems.
While many of these materials have high hydrogen storage capabilities, they are also characterized by less favorable characteristics such as pyrophoricity and water reactivity.
The authors of this research have performed experimental and modeling efforts to predict the consequences of these less-favorable characteristics during accident scenarios involving reactive solid hydrogen storage materials.
Using both fault tree (FT) and experimentally validated numerical methods, the failure mechanisms and possible outcomes of storage system accident scenarios are described Possible outcomes include system over-temperate, vessel overpressure, and loss of containment if un-mitigated.
Link:
https://www.ieahydrogen.org/tasks-reports/#17-17-task-reports-p2
The importance of integrating E-LCA and S-LCA for the deployment of hydrogen technologies is discussed, with an emphasis on the challenges of conducting S-LCA for low Technology Readiness Level (TRL) hydrogen production pathways. The study concludes that combining E-LCA and S-LCA results for product systems and processes can significantly contribute to the United Nations' seventeen Sustainable Development Goals (SDGs) and align with the UN's Environmental, Social, and Governance (ESG) dimensions. Incorporating this combined framework into ESG reporting enhances the accuracy, transparency, and credibility of sustainability disclosures, thereby supporting improved decision-making and stakeholder engagement. Key recommendations from the research include: a) the necessity of integrating E-LCA with S-LCA to provide comprehensive sustainability assessments and to identify environmental and social hotspots across hydrogen production value chains, and b) recognizing that sustainable hydrogen deployment must be driven by environmental and economic benefits, as well as social responsibility, worker well-being, labor rights, and occupational health and safety, among other important social indicators.
Keywords: Environmental hotspots; social hotspots; E-LCA; S-LCA; SHDB; PSILCA
Note: Interested readers can find more details in Khalil’s (1997) doctoral dissertation in management science (Sc.D.).
Unsurprisingly, the expected electricity price wars, battles for survival, and tremendous pressure exerted by big customers for rate reliefs are driving the electric utilities to sign bilateral agreements and energy deals with their major wholesale customers (commercial and industrial) in order to maintain the utility's market share and main sources of revenue. For example, Michigan investor-owned utility (IOU) company has lowered the electricity rates for its 9,000 industrial customers by 4.2% and a handful of large companies such as General Motors
(GM) have already received 10-20% rate cuts. However, the inevitable by-product of employing
this business practice is about 8.2% rates rise for Michigan 1.4 million captive customers
(residential and small business customers) to make up for the lost revenue from large
electricity customers. Furthermore, state regulators (viz., Department of Public Utility Commission ‘DPUC’) realize the benefits of maintaining in-state economic vitality and recognize utilities' fear of losing major revenues and the threat of the so-called "stranded investments" for utilities with nuclear assets. In response, unsurprisingly, regulators at the state level (viz., DPUC’) tend to bless these special electric rate contracts. While such deals provide the customers with discounted electricity rates, it ensures that the customer would buy most of its electricity needs from the utility rather than self-generating (for example, building its own gas-fired generator that supplies electricity and steam) or buying cheaper electricity from other competitors such as the Independent Power Producers (IPPs) and out-of-state utilities (under the so-called electricity retail wheeling schemes and transactions).
In this research, a hypothetical scenario of the bilateral agreements between an electric
utility and a wholesale price-sensitive customer is discussed. This scenario is simulated using Powersim system dynamics (SD) software to model this scenario development with complex dynamics using causal feedback loops, accumulation of flows into stocks and time delays. Also discussed are the short-term gains and unintended long-term consequences of potential strategic options such as offering premium / discount periods and lowering electricity production costs to make up for the price differentials.
Yehia F. Khalil1 and Tom Elicson2
Abstract
The authors of this research used the Modular Accident Analysis Programs MAAP 4.0.2 and MAAP4-DOSE to calculate the revised source term for regulatory applications in three parts as follows: 1) Source term release to containment, which reflects the likelihood of its occurrence, 2) Fission product distribution and removal in containment, and 3) Source term release to the environment including off-site dose calculations.
All three of these calculations were performed in a single, integrated analysis with MAAP 4 for the dominant core-melt sequences identified in the Millstone IPE.* Such calculations have numerous regulatory applications, including the relaxation or allowable leakage limits, the relaxation of primary and secondary containment isolation requirements, and revisions to surveillance specifications for safety-related equipment.
Reference
*Khalil, Y. F. (1992). Millstone Unit 1 Individual Plant Examination (IPE) for Severe accident Vulnerabilities. Northeast Utilities, NUSCO Report # 174, 1992.
____________
1 Engineering Manager, Probabilistic Risk Assessment, Northeast Utilities, Berlin, CT
Dr. Yehia F. Khalil is the corresponding author. [email protected]
2 Fauske & Associates (FAI), IL.
Y. F. Khalil, Ph.D., Sc.D.
Engineering Manager, Probabilistic Risk Assessment (PRA)
Millstone Nuclear Power Station, Waterford, CT, USA
Abstract
The U.S. Nuclear Regulatory Commission’s (NRC) generic letter GL 88-20 on ‘Severe Accident Vulnerabilities’ required the U.S. nuclear licensees with BWR and Mark-I containment units to assess potential containment failure mechanisms including drywell liner failure by melt-through.
The interest in this phenomenology surfaced after several research efforts at U.S. national laboratories (BNL and ORNL) predicted that ex-vessel molten corium attack on drywell liner could be a dominant failure mode and, therefore, would be a major concern to public safety.
The author of this theoretical study performed deterministic (using MAAP severe accident simulation program) and probabilistic analyses (using fault tree and event tree analyses) to assess the vulnerability of Millstone Unit 1 to drywell liner to melt-through and creep-rupture should it come in direct contact with molten corium. Accident management strategies were recommended to reduce drywell liner vulnerability to melt-through, mitigate the severity of radionuclide releases (given a liner melt-through), and reduce the likelihood of combustible gas burns in the auxiliary building, given a liner melt-though. Finally, potential accident management concerns were identified.
The insights gained from the Individual Plants Examinations (IPEs) for MP-1 and EF-2 are also discussed in this work. For the most part, this comparative theoretical study is confined to the Back-End, viz., Level-II Probabilistic Risk Assessment (PRA), analysis of the IPE.
References:
Khalil, Y.F. (1992). Millstone Unit 1 Individual Plant Examination for Severe Accident Vulnerabilities, Northeast Utilities, NUSCO 174, March 1992.
Solid-state hydrogen storage materials are a high-energy density option for a variety of hydrogen fuel cell applications including forklifts, automobiles, and portable battery replacement systems.
While many of these materials have high hydrogen storage capabilities, they are also characterized by less favorable characteristics such as pyrophoricity and water reactivity.
The authors of this research have performed experimental and modeling efforts to predict the consequences of these less-favorable characteristics during accident scenarios involving reactive solid hydrogen storage materials.
Using both fault tree (FT) and experimentally validated numerical methods, the failure mechanisms and possible outcomes of storage system accident scenarios are described Possible outcomes include system over-temperate, vessel overpressure, and loss of containment if un-mitigated.
The objectives include: 1) Demonstrating the importance of integrating social metrics with environmental impact assessments, 2) Supporting policymakers and stakeholders in holistic evaluations of green H2 technologies, 3) Establishing a framework to support H2 technology codes and standards such as ISO/TS 19870, 4) Facilitating sustainability certification and commercialization of low-carbon H2 technologies, and 5) Identifying social bottlenecks that hinder the diffusion of H2 technologies in the energy market.
The research will employ selected case studies to demonstrate how the combined E-LCA and S-LCA framework can identify environmental and social hotspots. It will also perform SWOT analyses of green H2 deployments and provide actionable insights and recommendations to policy decision-makers and stakeholders. The study will use various tools such as Aspen HYSYS for process simulation, and LCA platforms like GaBi, SimaPro, and OpenLCA for assessing environmental and social impacts.
By collaborating with international stakeholders, including Argonne National Laboratory (ANL) in the USA and UN’s Life Cycle Initiative, this research aims to contribute to the global push for sustainable hydrogen technologies and support the achievement of the United Nations Sustainable Development Goals (SDGs).
This research will expand on the current author's research at the Imperial College of London, UK.
The basis for the diagnostic process is the "plant condition matrix" philosophy originated in the EPRI TBR. Briefly, the diagnosis involves an assessment of the plant RCS condition and the -Containment condition in a manner where the final "plant condition" is determined by one of eight possible end-states as follows:
An RCS condition of either badly damaged (BD) or ex-vessel (EX) coupled with a containment condition of either closed (CC), challenged (CH), impaired (I), or bypassed (B) yields a two-by-four matrix representing the eight potential plant conditions. Once a determination has been made W as to which plant condition exists, a unique hierarchy of critical actions (called Candidate High Level Actions), along with a logic for assessing the impact of taking the action, is presented to combat/mitigate the accident. The critical actions include such activities as, RCS injection, injection into steam generators, depressurization of steam generators, RCP restart, reactor cavity flooding, containment venting, containment spray and/or containment fan coolers actuation, and deprcssurization of the RCS. Assessment of these actions is based on a variety of well organized information in Phase 3, including statements of caution, competing effects with other actions, throttling criteria and suggestions for concurrent actions.
1) Task’s organizational structure, goal, and objectives.
2) Task’s activities and deliverables (by subtask).
3) New hydrogen safety expert joined IEA Hydrogen.
4) Task 37 website and Hydrogen Safety Journal initiation.
5) Proposed forward looking opportunities for Task 37.
6) Challenges for hydrogen-based industry.
7) Message for hydrogen safety stakeholders.
1) DOE 2025 technical targets for onboard hydrogen storage for light-duty vehicles (LDV).
2) DOE/UTRC contract on hydrogen storage materials reactivity and safety.
in Zaragoza, Spain, June 16, 2016.
1) Conduct a preliminary engineering analysis for a proposed hybrid CuO-CuSO4 plant with 1000 MW (equivalent to about 30,240 kg/hr hydrogen production rate):
1.1) Material and energy balances
1.2) Cost estimation of major equipment
2) Discuss the use of nuclear power as an economically viable option for supplying the net thermal energy requirements of this hybrid cycle
3) Assess the applicability of solar (power tower) option to provide the necessary thermal energy for the hybrid cycle
1) What effect does increasing the gain have on the system response?
2) What is meant physically by a system with a large gain?
3) What effect does decreasing the time constant have on the system response?
4) What is meant physically by a system with a small time constant?
5) Is it possible for a system to have a negative time constant? What is the expected behavior?
6) Is it possible for a system to have a negative gain? What is the expected behavior?
B) Calculate a first-order approximation for an unknown system.
C) Calculate a second-order approximation from an unknown system.
Chapter 1: Task goal and objectives
Chapter 2: Task member countries and organizational structure
Chapter 3: Task main achievements, deliverables, and safety knowledge dissemination
Chapter 4: Hydrogen safety and the path forward
Chapter 5: Task 37 key messages and recommendations
This technical report also has 3 appendices as follows:
Appendix A: Task 37 website
Appendix B: Hydrogen Safety Journal
Appendix C: Examples of Task 37 annual meetings
Task 37 provided the following key messages and recommendations for future hydrogen R&D activities:
1) Over its six-years duration, Task 37 provided key quantitative risk insights (both physics-based and probabilistic) to support the development of both new and revised hydrogen safety C&S (e.g., NFPA-2 and ISO standards).
2) Dr. Khalil emphasizes the importance of expanding the current scope of hydrogen safety beyond the hydrogen-powered light-duty electric vehicles (LDEV) application and H2 refueling stations. To this end, Dr. Khalil recommends expanding the scope of H2 safety to other applications such as maritime, commercial aviation (hybrid-electric & all-electric aircraft), power-to-gas (P2G), heavy-duty vehicles, trains, and H2 transport in long road tunnels and other confined-spaces such as garages. Accordingly, additional R&D efforts are needed to ensure the safety of emerging hydrogen-based technologies and associated infrastructures.
3) Bulk storage of hydrogen (whether as compressed gas or liquified) would require investigation of novel tanks design, materials selection, more robust risk mitigation and control methods, and H2 leakage detection devices.
4) Comprehensive safety-related research efforts are needed to address materials-compatibility issues associated with hydrogen.
5) Improved understanding of safety issues is needed with respect to separation distances (aka, setback distances or safety distances), underground and above-ground hydrogen storage, leakage of hydrogen from transport pipelines, injecting hydrogen gas in existing natural gas networks, risks associated with blending hydrogen with natural gas for domestic heating, etc.
6) Hydrogen production, transport & distribution, supply chains safety risks, infrastructure physical security and vulnerability assessment, as well as safety codes & standards continue to be central issues for achieving the desired economies of scale and reliable adoption of hydrogen-based technologies.
7) There is a need for harmonizing hydrogen safety codes & standards to remove (or at least lower) unnecessary regulatory barriers and to establish common standards that ensure safety in each stage of the hydrogen value chain. Achieving this goal will accelerate the deployment of at-scale hydrogen-based technologies.
As a concluding remark on what Task 37 of the Hydrogen Technical Collaboration Program (H2 TCP) has accomplished over the past six years, Dr. Khalil emphasizes that: We must continue to seize all emerging opportunities to demonstrate, via science-based methods, the safety of hydrogen-based technologies.
The author of this technical report is Dr. Y. F. Khalil, the Operating Agent (OA) and Manager of the Hydrogen Safety Task 37 (which started in January 2015 and ended in December 2021) of the International Energy Agency (IEA) headquartered in Paris, France.
Learning Objectives:
1) Understand fundamentals of Probabilistic Risk Assessment (PRA) methods and tools.
2) Understand the concepts of nuclear reactor safety regulations.
A) Research Profile (2008-09):
A.1) “Recovery of Pyridine and 3-Methylpyridine from Impurity Streams of Pyridine Manufacturing Plants.” Three student teams worked on this project. Faculty Adviser: Prof. Yehia Khalil.
A.2) “Coal to Methanol Conversion.” Faculty Adviser: Prof. Yehia Khalil.
B) Teaching Profile (2008-09):
CENG 412b, Chemical Engineering Laboratory.
Prof. Yehia Khalil.
W 12:00-4:00 Sc Meets RP (36)
Basic experiments in chemical engineering science, including interpretation, analysis, and modeling of experimental results. Typical experiments include liquid level control, convective heat transfer, electrophoresis of colloidal particles, surface tension, surface wettability measurements, particle sedimentation, microfiltration, and flow in porous media.
CENG 416b/ENVE 416b, Chemical Engineering Process Design.
Prof. Yehia Khalil.
T Th 7:00-8:15 p.m. (evening) QR, Sc Meets RP (0)
Study of the techniques for and the design of chemical processes and plants, applying the principles of chemical engineering and economics. Emphasis on flowsheet development and equipment selection, cost estimation and economic analysis, design strategy and optimization, safety and hazards analysis, and environmental and ethical considerations.
Prerequisites: CENG 301b and 411a.
CENG 480a, Chemical Engineering Process Control.
Prof. Yehia Khalil.
T Th 9:00-10:15 QR, Sc Meets RP (22)
Transient regime modeling and simulations of chemical processes. Conventional and state-space methods of analysis and control design. Applications of modern control methods in chemical engineering. Course work includes a design project. Prerequisite: ENAS 194a or b or permission of instructor.
ENAS 445a/ENVE445a, Environmental Risk Assessment.
Prof. Yehia Khalil.
For description, see under Environmental Engineering.
ENVE 416b/CENG 416b, Chemical Engineering Process Design.
Prof. Yehia Khalil.
For description, see under Chemical Engineering.
ENVE 445a/ENAS 445a, Environmental Risk Assessment.
Prof. Yehia Khalil.
W F 4-5.15 (37)
Fundamentals and applications of probabilistic risk assessment and management in the context of environmental issues. Focus on developing and applying probabilistic and deterministic models to quantify potential risks of industrial processes and support risk-based decisions that account for societal, environmental, and economic constraints. Case studies emphasize the importance of green energy sources, professional ethics, and public health and safety. Prerequisite: ENVE 120b or permission of instructor.
412b Chemical Engineering Laboratory (Prof. Yehia Khalil)
416b/ENVE 416b Chemical Engineering Process Design (Prof. Yehia Khalil)
480a Chemical Engineering Process Control (Prof. Yehia Khalil)
445a/ENAS 445a Environmental Risk Assessment (Prof. Yehia Khalil)
Prof. Khalil developed and taught the ERA course at Yale School of Engineering & Applied Science (SEAS) as well as at Yale School of Forestry & Environmental Studies (F&ES).
Prof. Yehia Khalil
Office: M8, Mason Lab
Chemical Engineering teaching Lab: m228
Mason Laboratory, 9 Hillhouse Ave
New Haven, CT 06520
USA
Course number: ENVE S105
School of Engineering & Applied Science (SEAS), Yale University
New Haven, CT 06520 USA
This course focuses on fundamental aspects of risk, using environmental and health risks in the public and private sectors as its primary examples, and is designed to provide students with a broad understanding of the value of risk assessment and risk management (RA/RM) in the environmental engineering profession. Students learn how to identify potential hazards, quantify associated risks using probabilistic methods, and incorporate both probabilistic and deterministic results from environmental risk assessment (ERA) into the decision-making process after taking into consideration risk-to-risk trade-offs and societal, environmental, and economic consequences. Students learn how to apply methods and tools for qualitative and quantitative risk assessment (QLRA and QRA).
1) Wet FGS Systems / Scrubbers / Absorbers: Reagent: Ca(OH)2 slurry in counter current flow with the flue gas. Forced oxidation to convert CaSO3 to CaSO4 Dewatering / vacuum filters to form solid cake of CaSO4.2H2O
2) Spray Dryer Absorber (SDA): Reagent: Ca(OH)2 slurry Dry product, CaSO3.1/2H2O in removed by a BH or ESP downstream from the SDA
3) Post-Furnace Dry Sorbent Injection (DSI) Reagent: CaO
This lecture notes is part of Prof. Khalil's Air Pollution Control (APC) course at Yale SEAS and Yale F&ES.
Prof. Khalil taught the Chemical Engineering Process Design Course (CENG-416) for over 10 years at Yale University, New Haven, CT 06520 USA
The lecture covers the following topics:
1) Source of wind energy.
2) Environmental impact and public acceptance.
3) Failure mechanisms of wind turbines.
4) Kinetic energy (KE) of wind.
5) Types of wind turbines (WT): horizontal and vertical blade designs.
6) Dependence of the rotor’s power coefficient Cp on the tip-speed ratio
7) Power output of a wind turbine.
8) Greenhouse gases emissions and WT life cycle analysis (LCA)
9) Wind farms.
10) Economics of wind power.
Professor Khalil's invited lecture at Yale School of Forestry & Environmental Studies (F&ES), Yale University, USA
Lecture content:
1) NOx emission control
• At-the-source control technologies
• Down-the-pipe control technologies
2) SO2 emission control
• Options: scrub, switch, trade allowances or retire plant
• Other options
• Down-the-pipe technologies: wet FGD, semi-dry FGD (SDA) and dry (DSI) FGD 3) Summary
NOx and SO2 Emissions Control Technologies and Regulatory Options (Invited lecture at Yale School of Forestry & Environmental Studies). Available from: https://www.researchgate.net/publication/315703696_NOx_and_SO2_Emissions_Control_Technologies_and_Regulatory_Options_Invited_lecture_at_Yale_School_of_Forestry_Environmental_Studies [accessed Mar 30, 2017].
Professor Khalil is a faculty member at the School of Engineering & Applied Science (SEAS) at Yale University and previously was the Engineering Manager of the Probabilistic Risk Assessment Department at the Millstone Nuclear Power Station in Waterford, CT, USA. Professor also lectured at MIT Summer Courses on Nuclear Safety and Nuclear Reactor Technologies
Strengths, Weaknesses, Opportunities, and Threats (SWOT) Analysis of Fission Nuclear Power for Electricity Generation. Available from: https://www.researchgate.net/publication/316250278_Strengths_Weaknesses_Opportunities_and_Threats_SWOT_Analysis_of_Fission_Nuclear_Power_for_Electricity_Generation [accessed Apr 19, 2017].
Course summary:
Kinetics and thermodynamics of chemical reactions of common air pollutants. The role of surface chemistry and photochemistry in air pollution. Engineering technologies available to control air pollutants discussed in conjunction with their chemistry.
Course prerequisite: ChE210a or permission of the course instructor.
The course involves a series of basic experiments in fluid dynamics, heat transfer, mass transfer, process control, separations, and chemical kinetics. The goal of each laboratory exercise is to acquire the necessary laboratory data to either solve a design problem or answer fundamental questions about the underlying chemical and physical phenomena. Prior to each laboratory sequence, you will review the relevant literature, decide what data you will need to collect, how the data should be reduced and plotted, and plan your experiments accordingly. After completing each laboratory sequence, you will prepare a report detailing your experimental findings and your solution to the design problem and/or answers to the fundamental questions.
While APC is an elective course for the Yale Chemical Engineering Students, it is a core course for the Environmental Engineering Students.
Prof. Khalil's APC course is an extension of a previous graduate-level course (Environmental Control Technologies) that he developed and taught at Yale University for several years.
Course developed and taught by Professor Yehia Khalil at Yale University.
Abstract:
A look at the role of green energy — such as solar thermal, solar photovoltaic, wind, biofuel, hydro, geothermal, and nuclear power; ocean thermal energy conversion; and harvesting of tidal power — in mitigating the impact of greenhouse gases and global warming.
Dr. Y. F. Khalil served as the Hydrogen Safety Task Operating Agent (OA) and Manager for 6 years (two consecutive terms).
In this capacity, Dr. Khali led a team of hydrogen safety experts from countries around the world including USA, Canada, France, UK, Finland, Norway, Italy, the Netherland, China, and Japan.
His citation:
"For international recognition as an expert in risk assessment, product reliability & safety; for leading the International Energy Agency’s Hydrogen Technologies; for outstanding technical contributions as an inventor, co-designer of Carrier’s Microsteam Turbine Generator and Otis Electronic Safety Actuation; for innovative teaching in Environmental and chemical engineering at UConn and Yale."
URL: https://casemembers.wildapricot.org/Sys/PublicProfile/48518255/1086086
1) Coal conversion technology: Gasification
2) Targeted methanol production capacity: 5000MT/day
3) AA Grade methanol specifications:
>99.85% w/w methanol (dry basis)
<0.1% w/w water
<50 ppmw ethanol
Plant simulation is performed using Aspen HYSYS process simulation platform.
A system and method for performing time-dependent reliability prediction of a printed circuit board (PCB) embedded in a sensor that monitors the health (viz., performance) of operating equipment subject to different environmental stressors. The method includes developing a digital twin (DT) of the physical PCB, generating sensor data, and historical conditional data by the twining module, wherein the historical conditional data includes known failure data of one or more electronic components of the circuit board (CB) based on an internal condition or an external condition. The method further includes embedded physics-based reliability models informed by inputs from the sensor data and the historical conditional data, generating a real-time failure prediction signals based on the physics-based reliability models, and reporting the real-time failure prediction signal. The circuit board may include printed circuit board (PCBs), particularly additively-manufactured printed circuit boards (AM-PCBs).
http://environment.yale.edu/content/profiles/docs/yehia-khalil-publications.pdf?1441479062