Papers by Samantha L Clarke
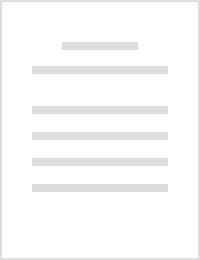
Landslides, 2019
A hazard assessment of submarine landslide-generated tsunami for the east Australian continental ... more A hazard assessment of submarine landslide-generated tsunami for the east Australian continental slope between Jervis Bay and Fraser Island is presented. Submarine landslides are present in water depths of approximately 400 to 3500 m along the entire length of continental margin, but are increasingly prevalent northward of Coffs Harbour without clustering at any particular water depth. Two hundred sixty individual submarine landslide scars that are greater than 1 km in width h ave been identified. Of these, 36 have been calculated to produce a tsunami flow depth equal to or greater than 5 m at the coastline for an assumed landslide downslope velocity of 20 ms−1. Landslides that are both thick (> 100 m) and wide (> 5 km) have the greatest potential to generate the largest coastal flow depths (> 10 m). The water depth of a landslide’s centre of mass strongly influences the onshore height of the tsunami’s surge with the larger events generated in shallower water depths (~ 500–1500 m). The maximum flow depth at the coastline is larger for thicker (50–250+ m) canyon landslides which occur on steeper slopes (> 4°), compared to thinner (< 50 m) plateau landslides which generally produce smaller tsunami. Maximum inundation distances and run-up heights of 1.6 km and 22 m respectively have been calculated for landslide velocities of 20 ms−1 and these values vary significantly depending on local coastal topography. There is no evidence for a submarine landslide large enough and young enough to have generated a Holocene age megatsunami for the east coast of Australia.
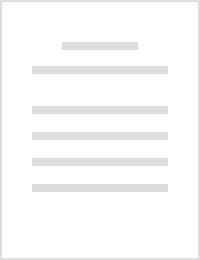
Geological Society, London, Special Publications, 477, SP477-11, 2018
Radiocarbon isotopic ages and sedimentological data are presented for material recovered from thr... more Radiocarbon isotopic ages and sedimentological data are presented for material recovered from three adjacent translational submarine landslides (YS1, YS2 and YS3) identified on the upper-continental slope offshore Yamba, New South Wales, Australia. The age data indicate that these three co-located upper-slope slides probably occurred independently of each other and not in a single, widespread regional-scale failure event.
Numerical estimates of the likely runout distances for slide blocks corresponding to the entire landslide scar volumes range between 10 and 27 km, and represent a ‘runout zone’ in which landslide blocks or debris might reasonably be expected to be located. There is no morphological evidence for large blocks or debris fields derived from two of the Yamba landslide scars within their identified runout zones (YS1 and YS2), suggesting these two failures involved complete disintegration of large slide blocks after failure or the removal of sediment from the landslide sites as grainflows or turbidites. In contrast, the third runout zone (YS3) presents good evidence of at least 12 slide blocks between 100 and 200 m in diameter, suggesting that they were shed as relatively small individual blocks or they were generated due to the dismemberment of a larger slab.
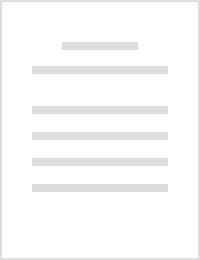
Sedimentological and accelerator mass spectrometry (AMS) 14C data provide estimates of the struct... more Sedimentological and accelerator mass spectrometry (AMS) 14C data provide estimates of the structure and age of five submarine landslides (»0.4–3 km3) present on eastern Australia’s continental slope between Noosa Heads and Yamba. Dating of the post-slide conformably deposited sediment indicates sediment accumulation rates between 0.017 m ka–1 and 0.2 m ka–1, which is consistent with previous estimates reported for this area. Boundary surfaces were identified in five continental slope cores at depths of 0.8 to 2.2 m below the present-day seafloor. Boundary surfaces present as a sharp colour-change across the surface, discernible but small increases in sediment stiffness, a slight increase in sediment bulk density of 0.1 g cm–3, and distinct gaps in AMS 14C ages of at least 25 ka. Boundary surfaces are interpreted to represent a slide plane detachment surface but are not necessarily the only ones or even the major ones. Sub-bottom profiler records indicate that: (1) the youngest identifiable sediment reflectors upslope from three submarine landslides terminate on and are truncated by slide rupture surfaces; (2) there is no obvious evidence for a post-slide sediment layer draped over, or burying, slide ruptures or exposed slide detachment surfaces; and (3) the boundary surfaces identified within the cores are unlikely to be near-surface slide surfaces within an overall larger en masse dislocation. These findings suggest that these submarine landslides are
geologically recent (<25 ka), and that the boundary surfaces are either: (a) an erosional features that developed after the landslide, in which case the boundary surface age provides a minimum age for the landslide; or (b) detachment surfaces from which slabs of near-surface sediment were removed during landsliding, in which case the age of the sediment above the boundary surface indicates the approximate age of landsliding. While an earthquake-triggering mechanism is favoured for the initiation of submarine landslides on the eastern Australian margin, further evidence is required to confirm this interpretation.
Morphologic characterisation of five distinct, eastern Australian upper continental slope submari... more Morphologic characterisation of five distinct, eastern Australian upper continental slope submarine landslides enabled modelling of their tsunami hazard. Flow depth, run-up and inundation distance has been calculated for each of the five landslides. Future submarine landslides with similar characteristics to these could generate tsunami with maximum flow depths ranging 5–10 m at the coastline, maximum run-up of 5 m and maximum inundation distances of 1 km.
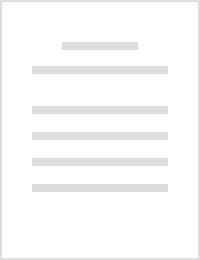
An investigation conducted aboard the RV Southern Surveyor (SS2013-V01) in January 2013 offshore ... more An investigation conducted aboard the RV Southern Surveyor (SS2013-V01) in January 2013 offshore east Australia collected regional bathymetric data for the continental margin of southern Queensland between Noosa Heads in the south and Indian Head, Fraser Island in the north. This newly mapped area presents a particularly steep portion of continental slope (5–10°) that presents numerous submarine landslides, including two ‘whole-of-slope’ features (the Wide Bay Canyon, and Inskip Slides). The slope is also dissected by three large submarine canyons offshore northern Fraser Island, Wide Bay, and Noosa Heads (i.e. the Fraser Canyons, the Wide Bay Canyon and the Noosa Canyon). Dredge and core samples were collected from slide scars in the northern, central, and southern areas of the bathymetric survey area. The initial examination of the area’s bathymetry, the core and dredge sample sedimentology, and determination of biostratigraphic ages for these sediment samples indicates that the larger submarine slides present in this study area have probably been shed from the slope since the late Pliocene and that canyon incision is currently active on this portion of the slope. In one case, canyon incision is partly responsible for generating slides due to undercutting and removal of the toe of the slope. Slope sediments are dominantly comprised of hemipelagic muds but also include grain-flows and turbidites comprised of shelf-derived sands and upper slope sediment that have abraided the slope muds. The results confirm previous work that indicates that this margin is in an active phase of deconstruction dominated by mass failure.
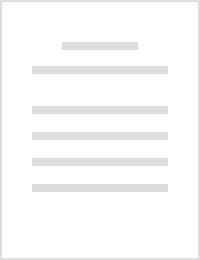
This paper examines the potential of tsunami inundation generated from two case study sites of su... more This paper examines the potential of tsunami inundation generated from two case study sites of submarine mass failures on the New South Wales coast of Australia. Two cases are investigated: the Bulli Slide and the Shovel Slide. Both slides are located approximately 65 km southeast of Sydney and 60 km east of the township of Wollongong.
The Bulli Slide (~20 km3) and the Shovel Slide (~8 km3) correspond to the two largest identified erosional surface submarine landslides scars of the NSW continental margin (Glenn et al., 2008; Clarke, 2014) and represent examples of large to very large submarine landslide scars. Previous work on the east Australian margin (Clarke et al., 2014) and elsewhere (Harbitz et al., 2013) suggests that submarine landslides similar in size to the Bulli and Shovel Slides are volumetrically large enough and occur at shallow enough water depths (400-2500 m) to generate substantial tsunamis that could cause widespread damage on the east Australian coast and threaten coastal communities (Burbidge et al., 2008; Clarke, 2014; Talukder and Volker, 2014).
Currently, the tsunamogenic potential of these two slides has only been investigated using 2D modelling (Clarke, 2014) and to date it has been difficult to establish the onshore tsunami surge characteristics for the submarine landslides with certainty.
To address this knowledge gap, coastal inundation resulting from these two mass failure events was investigated using a three-dimensional model (ANUGA) that predicts water flow resulting from natural hazard events such as tsunami (Nielsen et al., 2005). The ANUGA model solves the two-dimensional shallow water wave equations and accurately models the process of wetting and drying thus making it ideal for simulating inundation due to tsunami. The model generates a surface wave profile based on the dimensions of the submarine mass failure event using the method of Ward et al. (2011). Inundation maps are shown for these two slides and sensitivity analysis is conducted to identify the characteristics of the slides that are most influential on inundation areas and depths.
AGU Fall Meeting …, 2010
A large number of submarine landslides were identified on the continental slope on the Eastern Au... more A large number of submarine landslides were identified on the continental slope on the Eastern Australian margin during voyages aboard the RV Southern Surveyor in 2008. Preliminary sedimentological analysis as well as geotechnical and biostratigraphic data ...
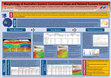
Advances in Natural and Technological Hazards Research, 2013
a a Submarine landslides can damage seabed infrastructure, cause subsidence of coastal land, and ... more a a Submarine landslides can damage seabed infrastructure, cause subsidence of coastal land, and generate tsunamis [1]. Examples of large submarine landslide generated tsunamis include the 1929 Grand Banks' event [2], the 1946 Scotch Cap Alaska event [3], and the 1998 Aitape Papua New Guinea event [4]. Submarine landslide generated tsunami are not as well understood as those associated with large earthquakes and consequently present a significant but poorly-quantified hazard [5]. The eastern Australia coast is potentially vulnerable to tsunamis due to the population concentration (~85%) and critical infrastructure within 50 km of the coast [6]. However, there has been little reason to suspect a local source for the generation of tsunami on the east Australian coastline. The identification of relatively recent, abundant submarine landslide scars has changed this perception [7][8][9] and established that submarine landsliding should be considered a common and ongoing characteristic of this passive continental margin [8,10].
A large number of submarine landslides were identified on the continental slope on the Eastern Au... more A large number of submarine landslides were identified on the continental slope on the Eastern Australian margin during voyages aboard the RV Southern Surveyor in 2008. Preliminary sedimentological analysis as well as geotechnical and biostratigraphic data determined for mid-slope dredge samples are reported. The dredge samples are normally-consolidated, calcareous sandy-muds of Neogene age and were recovered from submarine scarps located
Although the continental margins around Australia are themselves passive, they are surrounded by ... more Although the continental margins around Australia are themselves passive, they are surrounded by the Pacific Ring of Fire characterized by a great many earthquakes, tsunamis and submarine landslides. The Earthquake Catalogue of Geo-science Australia shows that in Australia earthquakes with a magnitude 5.5+ occur on average every two years with the potential of a disastrous earthquake of magnitude 6 or
Books by Samantha L Clarke
In book: Introduction to Natural Hazards: An Australasian Perspective, Edition: 1, Chapter: Lands... more In book: Introduction to Natural Hazards: An Australasian Perspective, Edition: 1, Chapter: Landslides, Publisher: Cambridge University Press, Editors: James Goff, Chris de Freitas
Thesis Chapters by Samantha L Clarke
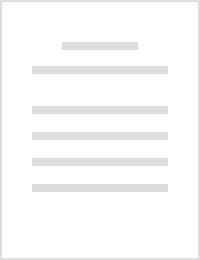
This study documents the causes of river channel change on the Hawkesbury-Nepean River in the Ric... more This study documents the causes of river channel change on the Hawkesbury-Nepean River in the Richmond Bridge area and investigates the natural and anthropogenic contributions to bank failure in the region. An historical aerial photograph analysis over the period 1947 to 2007 has been placed in the context of the long-term geological evolution of the channel and used to identify the timing, location and critical factors involved in bank collapse. This information has then been used to constrain geomechanical bank stability models which evaluate the factors responsible for the bank failures. Widespread collapse of the Nepean’s banks began in the early 1950s and continued until the late 1970s. The timing of the collapse coincides with a substantial increase in both the magnitude and frequency floods due to climatic fluctuation. The location of rotational mass failures in the Richmond Bridge area is confined exclusively to the left banks of the channel. The geomechanical analysis clearly demonstrates that two critical thresholds must be breached for large failures to occur. Firstly, the geometry of the banks needs to be relatively steep. The extensive failures of the left bank are a direct result of the bank slope (>25°). The steeper left banks experience massive circular failures between the 1950s to the late 1970s, while the less steep right banks opposite (<20°) do not present any visible evidence of failure from 1947 to the present (2007). Secondly, saturation of the soil mass and subsequent rapid drawdown are necessary to trigger bank failure; conditions which apply just after flooding (Morgenstern 1963; Docker and Hubble 2001). In contrast to other studies on the Hawkesbury-Nepean System (Hubble 2001; Hubble 2004), the density of bank vegetation is a relatively minor factor in determining the overall stability of river banks in the study area – increasing vegetation density and tree-root reinforcement of the soil mass would not have been sufficient to prevent the failure of the Richmond Bridge banks that did fail during the 1950’s, 1960’s and 1970’s Similarly, these bank collapses occurred before the extensive in-channel dredging and sand mining that took place in the 1980s; therefore it follows that local construction material extraction was not a causal factor for bank failure either. In addition, three conflicting river morphology models were evaluated to assess their ability to establish the causes of bank failure and channel change in the Richmond Bridge area. These are: 1) the FDR-DDR climatic model of Warner and Erskine; 2) the anthropogenic influence model of Brierley and co-workers; and 3) the intermediate model of Hubble and co-workers. These results call into question the role of devegetation as a major cause of bank failure and support the climatic cycling in the FDR-DDR model as the dominate trigger of channel change. While they do contribute necessary causal factors, neither the FDR-DDR model nor the anthropogenic influence model can, in isolation, explain the observed bank erosion, at least in this area. The geometry of the banks was the key determinant causing bank collapse in the area, in combination with the onset of the 1949-1991 FDR. It is suspected that channel change during the previous wet period (1850s-1902) left the banks in a condition predisposed to failure and that longer term geological change may also contribute. The incision of the Hawkesbury-Nepean channel through the floodplain and into the underlying geology has most likely resulted in over steepened left banks. The combination model of Hubble and coworkers therefore provides a better explanation to describe bank failure on the Nepean River. It brings together the important aspects of the FDR-DDR model and the anthropogenic influence model, while also demonstrating quantitatively how a particular bank will behave.
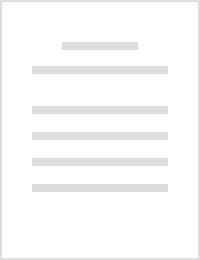
Stable continental margins experience submarine landslides relatively frequently and some of the ... more Stable continental margins experience submarine landslides relatively frequently and some of the largest slides on record have been shed from these relatively passive terrains. Despite this fact and the obvious accompanying tsunami hazard, slides from passive margins such as Australia are poorly understood when compared to other settings, such as the flanks of volcanic islands, active subduction-zone margins and submarine fans associated with large river deltas. This work presents an investigation into the submarine landslides occurring along eastern Australia’s upper continental margin, with a focus on investigating the causes, timing, and mechanisms responsible for these features. It has focused on analysing gravity core samples and interpreting of high-resolution multibeam and subbottom profiles. The age, morphology, composition, and origin of particular submarine landslides on the eastern Australian upper continental margin offshore New South Wales/Queensland has been described and, for the first time, the mechanical characteristics of sediments from the eastern Australian upper continental slope has been presented. The hazard posed by these submarine landslides has also been evaluated by investigating their potential to generate tsunamis along this margin.
Visually identified transition surfaces (boundary surfaces) are identified in five cores within, or adjacent to, five submarine landslides at depths of 0.8 to 2.2 meters below the present-day seafloor, which separate looser material from stiffer more compacted material. These boundary surfaces show distinct gaps in AMS 14C ages of at least 25 ka. Subbottom profiles across submarine landslides indicate that the youngest identifiable seismic reflectors located upslope of three slides terminate on and are truncated by slide rupture surfaces and that the studied slides are geologically recent. There is no obvious evidence in the subbottom profiles for a post-slide sediment layer draped over or otherwise burying slide ruptures or exposed slide detachment surfaces. This suggests that these submarine landslides are geologically recent, and that the boundary surfaces are either: a) erosional features that developed after the occurrence of the landslide in which case the boundary surface age provides a minimum age for landslide occurrence or b) detachment surfaces from which slabs of near-surface sediment were removed during landsliding in which case the age of the sediment above the boundary surface indicates approximately when landsliding occurred. While an earthquake triggering mechanism is favoured for the initiation of submarine landslides on the eastern Australian margin, this causal mechanism cannot be conclusively demonstrated.
Geomechanical test data are presented for 12 gravity cores taken at sites from the upper slope (<1200 m) of the east Australian continental margin in or adjacent to five submarine landslide features. The sediment from the study area presents as remarkably similar to sediment collected from other sections of the margin e.g. offshore Sydney, and the older compacted mud layers from lower in the stratigraphic section. The sediment is characterised by high shear strengths, low clay content and high void ratios, and brittle behaviour. It seems a major earthquake, or toe erosion could have contributed to initiate slides in this region. The identified boundary surfaces are interpreted to represent detachment surfaces or slide plane surfaces. The soil properties of the sediments show no evidence of weak clay layers, although they do contain significant but relatively small amounts of clay (<25%). Compression testing indicates that the sediment above and below the boundary surface is apparently slightly overconsolidated. The friction angles of the sediments are in the range of 30o - 40o, so that conventional soil mechanics would suggest the slopes have high factors of safety. However, this is clearly not the case as slope failures are widespread. Slide surfaces have been identified by dating and it has been shown that these features are associated with relatively shallow slides (~5-10 m) and not the large slide features that are evident in the bathymetry. Triaxial tests have indicated a significant increase in the brittleness of the shear response with increasing vertical stress level (i.e. burial depth), and that below a depth of ~20 m the soil response will be compressive, leading to the build up of pore pressure when subjected to cyclic (earthquake) loading. This is thought to be significant in explaining why the slides have large thicknessess of 50 to 200 m. To date, no conclusive triggering mechanism has been identified for initiating submarine landslides in the region although the brittle nature of the sediments and grainsize distribution make the slopes susceptible to liquefaction during oscillatory shaking, favouring an initation mechanism related to seismic shaking.
Evidence of submarine landsliding is presented for the east Australian continental margin between Jarvis Bay and Fraser Island. Thirty-six submarine landslide along the eastern Australian continental margin are identified that had the potential to produce a tsunami flow depth >5 m at the coastline. Flow depths at the coast range from 3 to 38 m for blocks moving downslope with a landslide velocity of 20 ms-1. Thin (<100 m) and narrow (<5 km) landslides produce smaller tsunami, with coastal flow depths of <5 m, and thick (>100 m) and/or wide (>5 km) landslides generate coastal flow depths of 5-10+ m. The combination of both thick and wide landslides had the greatest potential to generate the largest coastal flow depths >10 m. Maximum inundation distances and run-up heights of 1.6 km and 22 m respectively are calculated for landslide velocities of 20 ms-1, but these values vary significantly depending on local coastal topography. The reoccurrence of submarine landslides with similar characteristics to those shed from the margin in the geologically recent past would therefore be expected to generate tsunami with maximum flow depths between five and twenty meters at the coastline, run-up of up to 20 m and inundation distances of up to 1.5 km. The number of tsunamogenic submarine landslides identified increases northward of Coffs Harbour, with the number and size of both past tsunamogenic submarine landslides being much greater offshore northern New South Wales and southern Queensland than in central and southern New South Wales. Tsunamogenic submarine landslide scars reported for central and southern New South Wales are not big enough to have shed a block able to generate a megatsunami similar to paleo-megatsunamis hypothesised for this section of margin.
The widespread occurrence of upper slope slides across the eastern Australian margin indicates that submarine sliding should be considered to be a common characteristic of this passive continental margin. Engineering properties (friction angle, cohesion, and unit weight) imply that the sediment forming the margin is reasonably strong and inherently stable and classical limit-equilibrium modeling indicates that submarine landslides should not be a common occurrence on the margin. This indicates that pre-conditioning trigger, or some other mechanism is required to destabilise the slope and enable failure. The most likely suspected processes include: 1) dramatic reduction of the shear strength of the sediments to extremely low values, possibly induced by creep or a build-up of pore-pressure; 2) long-term modification of the slope-geometry i.e., sedimentation on the head of the slope and/or erosion of the toe of the slope; and/or 3) seismic events large enough to trigger sediment liquefaction or a sudden increase of pore-fluid pressure.
Conference Presentations by Samantha L Clarke
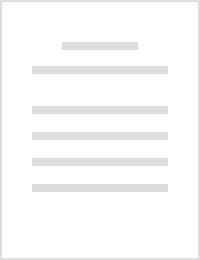
The 1.073 million km2 Murray-Darling River Basin (MDB) drains 14% of Australia’s landmass, incorp... more The 1.073 million km2 Murray-Darling River Basin (MDB) drains 14% of Australia’s landmass, incorporates Australia’s most economically important agricultural region, and presents one of Australia’s most important and contentious water security challenges. In this study we report the discovery of a hitherto unrecognised terminal palaeolake system ’Lake Mannum’ that developed during the middle to late Holocene, as evidenced by an extensive sequence of laminated muds. The deposit contains grey coloured laminae rich in Fe and smectite, and olive-black coloured laminae K and illite rich, representing high discharge events from both the Darling and Murray River catchments respectively, providing a high-resolution proxy record of MDB hydroclimatic variability during the Holocene. Given the strong influence of major oceanic-atmospheric synoptic circulation features (ENSO, IOS-SAM, IPO, IDO) over the river system, variability in MDB discharge and delivery of suspended sediment flux to the continental shelf have been used as proxy indicators for southeastern Australian palaeoclimate during the Holocene. The existence of a lake system at the terminus of the MDB suggests that discharge of terrigenous sediment to the Southern Ocean was strongly suppressed during this time, meaning that Holocene climate reconstructions for SE Australia which rely on the marine sediment record require re-evaluation.
Uploads
Papers by Samantha L Clarke
Numerical estimates of the likely runout distances for slide blocks corresponding to the entire landslide scar volumes range between 10 and 27 km, and represent a ‘runout zone’ in which landslide blocks or debris might reasonably be expected to be located. There is no morphological evidence for large blocks or debris fields derived from two of the Yamba landslide scars within their identified runout zones (YS1 and YS2), suggesting these two failures involved complete disintegration of large slide blocks after failure or the removal of sediment from the landslide sites as grainflows or turbidites. In contrast, the third runout zone (YS3) presents good evidence of at least 12 slide blocks between 100 and 200 m in diameter, suggesting that they were shed as relatively small individual blocks or they were generated due to the dismemberment of a larger slab.
geologically recent (<25 ka), and that the boundary surfaces are either: (a) an erosional features that developed after the landslide, in which case the boundary surface age provides a minimum age for the landslide; or (b) detachment surfaces from which slabs of near-surface sediment were removed during landsliding, in which case the age of the sediment above the boundary surface indicates the approximate age of landsliding. While an earthquake-triggering mechanism is favoured for the initiation of submarine landslides on the eastern Australian margin, further evidence is required to confirm this interpretation.
The Bulli Slide (~20 km3) and the Shovel Slide (~8 km3) correspond to the two largest identified erosional surface submarine landslides scars of the NSW continental margin (Glenn et al., 2008; Clarke, 2014) and represent examples of large to very large submarine landslide scars. Previous work on the east Australian margin (Clarke et al., 2014) and elsewhere (Harbitz et al., 2013) suggests that submarine landslides similar in size to the Bulli and Shovel Slides are volumetrically large enough and occur at shallow enough water depths (400-2500 m) to generate substantial tsunamis that could cause widespread damage on the east Australian coast and threaten coastal communities (Burbidge et al., 2008; Clarke, 2014; Talukder and Volker, 2014).
Currently, the tsunamogenic potential of these two slides has only been investigated using 2D modelling (Clarke, 2014) and to date it has been difficult to establish the onshore tsunami surge characteristics for the submarine landslides with certainty.
To address this knowledge gap, coastal inundation resulting from these two mass failure events was investigated using a three-dimensional model (ANUGA) that predicts water flow resulting from natural hazard events such as tsunami (Nielsen et al., 2005). The ANUGA model solves the two-dimensional shallow water wave equations and accurately models the process of wetting and drying thus making it ideal for simulating inundation due to tsunami. The model generates a surface wave profile based on the dimensions of the submarine mass failure event using the method of Ward et al. (2011). Inundation maps are shown for these two slides and sensitivity analysis is conducted to identify the characteristics of the slides that are most influential on inundation areas and depths.
Books by Samantha L Clarke
Thesis Chapters by Samantha L Clarke
Visually identified transition surfaces (boundary surfaces) are identified in five cores within, or adjacent to, five submarine landslides at depths of 0.8 to 2.2 meters below the present-day seafloor, which separate looser material from stiffer more compacted material. These boundary surfaces show distinct gaps in AMS 14C ages of at least 25 ka. Subbottom profiles across submarine landslides indicate that the youngest identifiable seismic reflectors located upslope of three slides terminate on and are truncated by slide rupture surfaces and that the studied slides are geologically recent. There is no obvious evidence in the subbottom profiles for a post-slide sediment layer draped over or otherwise burying slide ruptures or exposed slide detachment surfaces. This suggests that these submarine landslides are geologically recent, and that the boundary surfaces are either: a) erosional features that developed after the occurrence of the landslide in which case the boundary surface age provides a minimum age for landslide occurrence or b) detachment surfaces from which slabs of near-surface sediment were removed during landsliding in which case the age of the sediment above the boundary surface indicates approximately when landsliding occurred. While an earthquake triggering mechanism is favoured for the initiation of submarine landslides on the eastern Australian margin, this causal mechanism cannot be conclusively demonstrated.
Geomechanical test data are presented for 12 gravity cores taken at sites from the upper slope (<1200 m) of the east Australian continental margin in or adjacent to five submarine landslide features. The sediment from the study area presents as remarkably similar to sediment collected from other sections of the margin e.g. offshore Sydney, and the older compacted mud layers from lower in the stratigraphic section. The sediment is characterised by high shear strengths, low clay content and high void ratios, and brittle behaviour. It seems a major earthquake, or toe erosion could have contributed to initiate slides in this region. The identified boundary surfaces are interpreted to represent detachment surfaces or slide plane surfaces. The soil properties of the sediments show no evidence of weak clay layers, although they do contain significant but relatively small amounts of clay (<25%). Compression testing indicates that the sediment above and below the boundary surface is apparently slightly overconsolidated. The friction angles of the sediments are in the range of 30o - 40o, so that conventional soil mechanics would suggest the slopes have high factors of safety. However, this is clearly not the case as slope failures are widespread. Slide surfaces have been identified by dating and it has been shown that these features are associated with relatively shallow slides (~5-10 m) and not the large slide features that are evident in the bathymetry. Triaxial tests have indicated a significant increase in the brittleness of the shear response with increasing vertical stress level (i.e. burial depth), and that below a depth of ~20 m the soil response will be compressive, leading to the build up of pore pressure when subjected to cyclic (earthquake) loading. This is thought to be significant in explaining why the slides have large thicknessess of 50 to 200 m. To date, no conclusive triggering mechanism has been identified for initiating submarine landslides in the region although the brittle nature of the sediments and grainsize distribution make the slopes susceptible to liquefaction during oscillatory shaking, favouring an initation mechanism related to seismic shaking.
Evidence of submarine landsliding is presented for the east Australian continental margin between Jarvis Bay and Fraser Island. Thirty-six submarine landslide along the eastern Australian continental margin are identified that had the potential to produce a tsunami flow depth >5 m at the coastline. Flow depths at the coast range from 3 to 38 m for blocks moving downslope with a landslide velocity of 20 ms-1. Thin (<100 m) and narrow (<5 km) landslides produce smaller tsunami, with coastal flow depths of <5 m, and thick (>100 m) and/or wide (>5 km) landslides generate coastal flow depths of 5-10+ m. The combination of both thick and wide landslides had the greatest potential to generate the largest coastal flow depths >10 m. Maximum inundation distances and run-up heights of 1.6 km and 22 m respectively are calculated for landslide velocities of 20 ms-1, but these values vary significantly depending on local coastal topography. The reoccurrence of submarine landslides with similar characteristics to those shed from the margin in the geologically recent past would therefore be expected to generate tsunami with maximum flow depths between five and twenty meters at the coastline, run-up of up to 20 m and inundation distances of up to 1.5 km. The number of tsunamogenic submarine landslides identified increases northward of Coffs Harbour, with the number and size of both past tsunamogenic submarine landslides being much greater offshore northern New South Wales and southern Queensland than in central and southern New South Wales. Tsunamogenic submarine landslide scars reported for central and southern New South Wales are not big enough to have shed a block able to generate a megatsunami similar to paleo-megatsunamis hypothesised for this section of margin.
The widespread occurrence of upper slope slides across the eastern Australian margin indicates that submarine sliding should be considered to be a common characteristic of this passive continental margin. Engineering properties (friction angle, cohesion, and unit weight) imply that the sediment forming the margin is reasonably strong and inherently stable and classical limit-equilibrium modeling indicates that submarine landslides should not be a common occurrence on the margin. This indicates that pre-conditioning trigger, or some other mechanism is required to destabilise the slope and enable failure. The most likely suspected processes include: 1) dramatic reduction of the shear strength of the sediments to extremely low values, possibly induced by creep or a build-up of pore-pressure; 2) long-term modification of the slope-geometry i.e., sedimentation on the head of the slope and/or erosion of the toe of the slope; and/or 3) seismic events large enough to trigger sediment liquefaction or a sudden increase of pore-fluid pressure.
Conference Presentations by Samantha L Clarke
Numerical estimates of the likely runout distances for slide blocks corresponding to the entire landslide scar volumes range between 10 and 27 km, and represent a ‘runout zone’ in which landslide blocks or debris might reasonably be expected to be located. There is no morphological evidence for large blocks or debris fields derived from two of the Yamba landslide scars within their identified runout zones (YS1 and YS2), suggesting these two failures involved complete disintegration of large slide blocks after failure or the removal of sediment from the landslide sites as grainflows or turbidites. In contrast, the third runout zone (YS3) presents good evidence of at least 12 slide blocks between 100 and 200 m in diameter, suggesting that they were shed as relatively small individual blocks or they were generated due to the dismemberment of a larger slab.
geologically recent (<25 ka), and that the boundary surfaces are either: (a) an erosional features that developed after the landslide, in which case the boundary surface age provides a minimum age for the landslide; or (b) detachment surfaces from which slabs of near-surface sediment were removed during landsliding, in which case the age of the sediment above the boundary surface indicates the approximate age of landsliding. While an earthquake-triggering mechanism is favoured for the initiation of submarine landslides on the eastern Australian margin, further evidence is required to confirm this interpretation.
The Bulli Slide (~20 km3) and the Shovel Slide (~8 km3) correspond to the two largest identified erosional surface submarine landslides scars of the NSW continental margin (Glenn et al., 2008; Clarke, 2014) and represent examples of large to very large submarine landslide scars. Previous work on the east Australian margin (Clarke et al., 2014) and elsewhere (Harbitz et al., 2013) suggests that submarine landslides similar in size to the Bulli and Shovel Slides are volumetrically large enough and occur at shallow enough water depths (400-2500 m) to generate substantial tsunamis that could cause widespread damage on the east Australian coast and threaten coastal communities (Burbidge et al., 2008; Clarke, 2014; Talukder and Volker, 2014).
Currently, the tsunamogenic potential of these two slides has only been investigated using 2D modelling (Clarke, 2014) and to date it has been difficult to establish the onshore tsunami surge characteristics for the submarine landslides with certainty.
To address this knowledge gap, coastal inundation resulting from these two mass failure events was investigated using a three-dimensional model (ANUGA) that predicts water flow resulting from natural hazard events such as tsunami (Nielsen et al., 2005). The ANUGA model solves the two-dimensional shallow water wave equations and accurately models the process of wetting and drying thus making it ideal for simulating inundation due to tsunami. The model generates a surface wave profile based on the dimensions of the submarine mass failure event using the method of Ward et al. (2011). Inundation maps are shown for these two slides and sensitivity analysis is conducted to identify the characteristics of the slides that are most influential on inundation areas and depths.
Visually identified transition surfaces (boundary surfaces) are identified in five cores within, or adjacent to, five submarine landslides at depths of 0.8 to 2.2 meters below the present-day seafloor, which separate looser material from stiffer more compacted material. These boundary surfaces show distinct gaps in AMS 14C ages of at least 25 ka. Subbottom profiles across submarine landslides indicate that the youngest identifiable seismic reflectors located upslope of three slides terminate on and are truncated by slide rupture surfaces and that the studied slides are geologically recent. There is no obvious evidence in the subbottom profiles for a post-slide sediment layer draped over or otherwise burying slide ruptures or exposed slide detachment surfaces. This suggests that these submarine landslides are geologically recent, and that the boundary surfaces are either: a) erosional features that developed after the occurrence of the landslide in which case the boundary surface age provides a minimum age for landslide occurrence or b) detachment surfaces from which slabs of near-surface sediment were removed during landsliding in which case the age of the sediment above the boundary surface indicates approximately when landsliding occurred. While an earthquake triggering mechanism is favoured for the initiation of submarine landslides on the eastern Australian margin, this causal mechanism cannot be conclusively demonstrated.
Geomechanical test data are presented for 12 gravity cores taken at sites from the upper slope (<1200 m) of the east Australian continental margin in or adjacent to five submarine landslide features. The sediment from the study area presents as remarkably similar to sediment collected from other sections of the margin e.g. offshore Sydney, and the older compacted mud layers from lower in the stratigraphic section. The sediment is characterised by high shear strengths, low clay content and high void ratios, and brittle behaviour. It seems a major earthquake, or toe erosion could have contributed to initiate slides in this region. The identified boundary surfaces are interpreted to represent detachment surfaces or slide plane surfaces. The soil properties of the sediments show no evidence of weak clay layers, although they do contain significant but relatively small amounts of clay (<25%). Compression testing indicates that the sediment above and below the boundary surface is apparently slightly overconsolidated. The friction angles of the sediments are in the range of 30o - 40o, so that conventional soil mechanics would suggest the slopes have high factors of safety. However, this is clearly not the case as slope failures are widespread. Slide surfaces have been identified by dating and it has been shown that these features are associated with relatively shallow slides (~5-10 m) and not the large slide features that are evident in the bathymetry. Triaxial tests have indicated a significant increase in the brittleness of the shear response with increasing vertical stress level (i.e. burial depth), and that below a depth of ~20 m the soil response will be compressive, leading to the build up of pore pressure when subjected to cyclic (earthquake) loading. This is thought to be significant in explaining why the slides have large thicknessess of 50 to 200 m. To date, no conclusive triggering mechanism has been identified for initiating submarine landslides in the region although the brittle nature of the sediments and grainsize distribution make the slopes susceptible to liquefaction during oscillatory shaking, favouring an initation mechanism related to seismic shaking.
Evidence of submarine landsliding is presented for the east Australian continental margin between Jarvis Bay and Fraser Island. Thirty-six submarine landslide along the eastern Australian continental margin are identified that had the potential to produce a tsunami flow depth >5 m at the coastline. Flow depths at the coast range from 3 to 38 m for blocks moving downslope with a landslide velocity of 20 ms-1. Thin (<100 m) and narrow (<5 km) landslides produce smaller tsunami, with coastal flow depths of <5 m, and thick (>100 m) and/or wide (>5 km) landslides generate coastal flow depths of 5-10+ m. The combination of both thick and wide landslides had the greatest potential to generate the largest coastal flow depths >10 m. Maximum inundation distances and run-up heights of 1.6 km and 22 m respectively are calculated for landslide velocities of 20 ms-1, but these values vary significantly depending on local coastal topography. The reoccurrence of submarine landslides with similar characteristics to those shed from the margin in the geologically recent past would therefore be expected to generate tsunami with maximum flow depths between five and twenty meters at the coastline, run-up of up to 20 m and inundation distances of up to 1.5 km. The number of tsunamogenic submarine landslides identified increases northward of Coffs Harbour, with the number and size of both past tsunamogenic submarine landslides being much greater offshore northern New South Wales and southern Queensland than in central and southern New South Wales. Tsunamogenic submarine landslide scars reported for central and southern New South Wales are not big enough to have shed a block able to generate a megatsunami similar to paleo-megatsunamis hypothesised for this section of margin.
The widespread occurrence of upper slope slides across the eastern Australian margin indicates that submarine sliding should be considered to be a common characteristic of this passive continental margin. Engineering properties (friction angle, cohesion, and unit weight) imply that the sediment forming the margin is reasonably strong and inherently stable and classical limit-equilibrium modeling indicates that submarine landslides should not be a common occurrence on the margin. This indicates that pre-conditioning trigger, or some other mechanism is required to destabilise the slope and enable failure. The most likely suspected processes include: 1) dramatic reduction of the shear strength of the sediments to extremely low values, possibly induced by creep or a build-up of pore-pressure; 2) long-term modification of the slope-geometry i.e., sedimentation on the head of the slope and/or erosion of the toe of the slope; and/or 3) seismic events large enough to trigger sediment liquefaction or a sudden increase of pore-fluid pressure.