Papers by Laurent Gernigon
Geophysical …, Jan 1, 2009
The Jan Mayen Fault Zone (JMFZ) is probably the most atypical expression of the Norwegian-Greenla... more The Jan Mayen Fault Zone (JMFZ) is probably the most atypical expression of the Norwegian-Greenland Sea and its complexity and profound nature are clearly mirrored in contrasting spreading configurations visible in the North Atlantic oceanic domain. In order to better constrain the structure and the tectonic evolution of the JMFZ and contiguous margins, a new aeromagnetic survey (JAS-05) was acquired
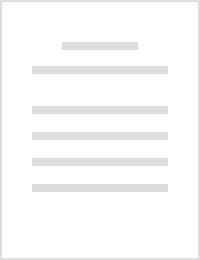
Gernigon, L., Blischke, A., Nasuti, A., Olesen, O., Sand, M. and Arnarson, T.S. 2013 Rift to drift evolution of the Jan Mayen Micro-continent and conjugate rifted margins: implication of new aeromagnetic surveys in the Norwegian-Greenland Sea We re-evaluate the structure and spreading evolution of the Norwegian-Greenland Sea and surroundi... more We re-evaluate the structure and spreading evolution of the Norwegian-Greenland Sea and surrounding volcanic (rifted) margins based on new high-resolution aeromagnetic surveys. The new dataset combined with seismic and gravity data allowed us to understand better the structure and evolution of the Nordic conjugate margin system from the rifting to the drifting stage. We particularly focus on the new JAS-12 aeromagnetic survey acquired between the Aegir Ridge and the Jan Mayen micro-continent, which was initially part of the Møre-Vøring-Greenland rift system. Combined with the previous NB-07 and JAS-05 surveys, our final compilation fully covers the continent-ocean transition and the whole oceanic spreading system from the Møre margin to the Jan Mayen micro-continent with high quality, high resolution and reliable magnetic data. The new dataset allowed us to get a new, consistent and precise interpretation of the magnetic polarity chrons and oceanic fractures, providing us now with t...
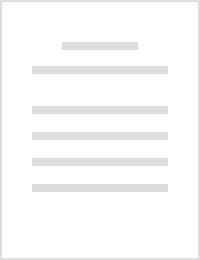
ABSTRACT We re-evaluate the structure and spreading evolution of the Norwegian-Greenland Sea and ... more ABSTRACT We re-evaluate the structure and spreading evolution of the Norwegian-Greenland Sea and surrounding volcanic (rifted) margins based on new high-resolution aeromagnetic surveys. The new dataset combined with long-offset seismic and gravity data allow us to have a better understanding of the structure and evolution of the conjugate margin systems in the Norwegian-Greenland Sea from the rifting to the drifting stage.We particularly focus on the new JAS-12 aeromagnetic survey acquired between the Aegir Ridge and the Jan Mayen micro-continent, which was initially part of the Møre-Vøring-Greenland rift system. Combined with the previous NB-07 and JAS-05 surveys, our final compilation fully covers the continent-ocean transition and the whole oceanic spreading system from the Møre margin to the conjugate Jan Mayen micro-continent with high quality, high-resolution and reliable magnetic data. The new dataset allowed a new, consistent and precise interpretation of the magnetic polarity chrons and oceanic fractures, providing the basis for more accurate rotation poles estimation, and better basin and crustal reconstructions between Norway, Greenland and the Jan Mayen micro-continent. This dataset allowed us to clarify the pre- and post-breakup configurations of the rift system and discuss the mechanisms involved during the onset of the two phases of breakup leading to the micro-continent formation. Our observations and models suggest that the pre-breakup rift system evolved through a significant Late Jurassic-Cretaceous thinning phase. This episode led to a significant thinning of the continental crust and an exhumation of pre-existing lower crust. However, we have not been able to identify and/or validate any clear domains of exhumed and denudated serpentinised mantle. The first Eocene breakup is mostly characterised by severe magmatism (sill, SDRS). Lithospheric/asthenospheric processes leading to rift localisation do not necessarily represent a continuum of lithospheric deformation with the precedent thinning system. Diking and disconnected lithospheric plumbing are proposed to explain the Eocene breakup. After the first phase of continental breakup, two major phases of spreading influenced the Norwegian–Greenland Sea. Phase I (from C24 to C21r, �54 to 49 Ma) marks the earliest phase of spreading, probably initiated in the central and outer part of the Møre Basin. During this period, the formation of overlapping systems and pseudo-fault development, indirectly influenced by the proto-margin segmentation, suggests the presence of additional micro-plates in the Norwegian-Greenland Sea. We also observed a significant change in the oceanic spreading system in the late Early Eocene. Based on observations from the surrounding areas, this supports a major and distinct tectonic and magmatic event in the Norwegian-Greenland Sea at around C21r (49-47.9 Ma), the beginning of a second phase. During Phase II, from C21r-C12 or possibly younger (48-<32 Ma) of the Norway Basin development, spreading rates decreased, spreading direction changed leading to the formation of unexpected N-S oriented oceanic fracture zones. Phase II probably coincides with the climax of extension and possibly local spreading that is suspected in the southern part of the Jan Mayen micro-continent forming a complex area of oceanic, transitional and continental fragments before its complete dislocation from East Greenland in Latest Oligocene.
Norsk Geologisk Tidsskrift, Jun 1, 2013
In order to better constrain the Cenozoic evolution of the Norwegian-Greenland Sea and contiguous... more In order to better constrain the Cenozoic evolution of the Norwegian-Greenland Sea and contiguous margins, a new aeromagnetic data (JAS-05 survey) was acquired in the Eastern part of the Jan Mayen Fracture Zone during autumn 2005. We present preliminary results and ...
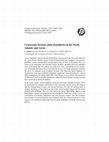
A new kinematic model from the pre-breakup to present day has been developed for the Arctic-North... more A new kinematic model from the pre-breakup to present day has been developed for the Arctic-North Atlantic region. Using potential field data (magnetic and gravity), published seismic interpretation and geological data we have re-interpreted the con-tinent ocean boundaries and transition zones. Seafloor spreading has been quantita-tively determined and new palaeo-age grids have been constructed for the oceanic area. Kinematic parameters have been used in the case of a triple junction to estimate the errors of continent ocean boundary location. The Oligocene plate boundary re-organization (and microcontinent formation) might have been precluded by various ridge propagations NE and possible SW of Jan Mayen microcontinent. The existence of additional (short lived) plate boundaries within the North Atlantic oceanic area has been tested using statistical methods. In the Arctic realm, several kinematic scenarios have been explored in order to bet-ter understand the complex architecture of...
The NE-Atlantic system represents the northward propagation of the Atlantic Ocean north of the Ch... more The NE-Atlantic system represents the northward propagation of the Atlantic Ocean north of the Charly Gibbs Fracture Zone (Fig. 1). This region includes both continental and oceanic domains and experienced a long and complex tectonic evolution since the Caledonian orogen.
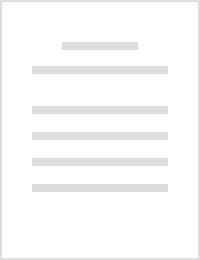
ABSTRACT The localisation of the deformation reflects the redistribution of stress into a particu... more ABSTRACT The localisation of the deformation reflects the redistribution of stress into a particular region which is rheologically weak. If an additional weaker zone is formed in an adjacent region, we expect the deformation to migrate towards this region. A relocation of the deformation could explain some geological phenomena including sedimentary basin migration and/or microcontinent formation. In this study, this mechanism is investigated by means of a two-dimensional finite element model where extension of the continental lithosphere is accommodated by progressive thinning of a rheologically weak region brought about by underplated mafic bodies (UPMB). A non-linear Maxwell viscoelastic rheology with a temperature-dependent viscosity controlled by power-law creep and an elastic - perfect plastic rheology model controlled by the Von Mises yield criterion are adopted for the ductile and brittle behaviours of rocks, respectively. The UPMB is assumed to be emplaced instantaneously without considering the nature of the melt generation and its upward migration. It is also assumed that the UPMB mostly develop just below the Moho which agree with refraction observations on volcanic margins. The generation of melts may require the asthenosphere to ascent into the shallower level in the lithosphere, which may imply that the deformation has already been localized. The most efficient way to obtain the reduction of the total lithosphere strength is to significantly weaken the shallower (colder) part of the lithosphere. Numerical experiments show that three modes of the deformation redistribution exist: 1) a shift-completed mode where the deformation localisation is completely redistributed into a different region, 2) a transition mode where the localisation is redistributed but extension is accommodated by thinning in two regions, and 3) a shift-failed mode where the localisation cannot be redistributed. The redistribution of the deformation localisation brought about by the UPMB depends on the initial rheological heterogeneity in the first deformed region and the configuration and timing of the UPMB's emplacement. However, the deformation localisation cannot be redistributed anymore if the emplacement of the UPMB occurs after the total stretching factor of the lithosphere in the firstly deformed region becomes greater than a critical value of ~ 2.25. The model behaviour presented in this study provides profitable implications for the sedimentary basin migration and/or the microcontinent formation. The redistribution of the deformation localisation that takes place before the stretching factor reaches the critical value in the firstly deformed region can be explained as the shift-completed or the transition mode. However, if the redistribution takes place after the firstly deformed region reaches the stretching factor greater than the critical value (i.e., the case of the microcontinent formation), we must only involve the transition mode, in which the localisation occurs in the two different regions, but one leads to the continental break-up earlier than the other.
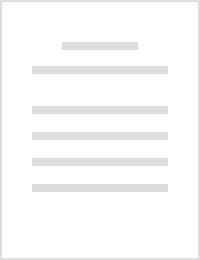
ABSTRACT It is important to understand a mechanism of the strain redistribution in order to progr... more ABSTRACT It is important to understand a mechanism of the strain redistribution in order to progress our understanding of sedimentary basin migration and/or continental-ribbon/micro-continent insulation. The change in the lithospheric strength due to thermal relaxation and/or replacement of weaker crust with stronger mantle has usually been considered as a possible mechanism that cases successive deformation to migrate to adjacent regions. This is, however, hardly applicable for an extensional process that can lead to continental break-up. In our previous studies (Yamasaki and Gernigon, Tectonophysics, 468, 169-184, 2009; J. Geol. Soc. Lond., 167, 961-971, 2010), the effect of underplated mafic bodies (UPMB) on the redistribution of extensional strain was examined quantitatively, in which UPMB is emplaced beneath a different region some time after the first rifting process has initiated. It was showed that there possibly exist three different modes of strain redistribution, 1) a shift-completed mode: the strain is completely shifted into a newly weakened region, 2) a transition mode: the strain is redistributed, but lithospheric extension is accommodated by thinning in two regions and 3) a shift-failed mode where the strain is not redistributed. The modes are dependent on the configuration of UPMB and on the initial rheological heterogeneity in the initially deformed region, but it becomes difficult for any UPMB to initiate the redistribution of the deformation once the stretching factor in the first deformed region exceeds a critical value. The general model behaviour can characterise the dynamic process of the strain migration, in terms of which the geological process observed during rifting and break-up is discussed in this study, providing an important implication for a typical rift/margin configuration that the shift-completed or transition mode can be applied for the regions where UPMB has been clearly observed (e.g., the Indian Ocean, the Norwegian-Greenland Sea, the Red Sea/Afar region, the Western Barents Sea and the Brazilian margin).
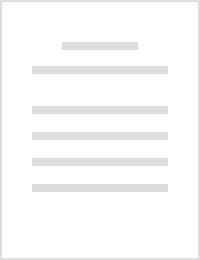
ABSTRACT We re-evaluate the structure and spreading evolution of the Norwegian-Greenland Sea and ... more ABSTRACT We re-evaluate the structure and spreading evolution of the Norwegian-Greenland Sea and surrounding volcanic (rifted) margins based on new high-resolution aeromagnetic surveys. The new dataset combined with long-offset seismic and gravity data allow us to have a better understanding of the structure and evolution of the conjugate margin systems in the Norwegian-Greenland Sea from the rifting to the drifting stage.We particularly focus on the new JAS-12 aeromagnetic survey acquired between the Aegir Ridge and the Jan Mayen micro-continent, which was initially part of the Møre-Vøring-Greenland rift system. Combined with the previous NB-07 and JAS-05 surveys, our final compilation fully covers the continent-ocean transition and the whole oceanic spreading system from the Møre margin to the conjugate Jan Mayen micro-continent with high quality, high-resolution and reliable magnetic data. The new dataset allowed a new, consistent and precise interpretation of the magnetic polarity chrons and oceanic fractures, providing the basis for more accurate rotation poles estimation, and better basin and crustal reconstructions between Norway, Greenland and the Jan Mayen micro-continent. This dataset allowed us to clarify the pre- and post-breakup configurations of the rift system and discuss the mechanisms involved during the onset of the two phases of breakup leading to the micro-continent formation. Our observations and models suggest that the pre-breakup rift system evolved through a significant Late Jurassic-Cretaceous thinning phase. This episode led to a significant thinning of the continental crust and an exhumation of pre-existing lower crust. However, we have not been able to identify and/or validate any clear domains of exhumed and denudated serpentinised mantle. The first Eocene breakup is mostly characterised by severe magmatism (sill, SDRS). Lithospheric/asthenospheric processes leading to rift localisation do not necessarily represent a continuum of lithospheric deformation with the precedent thinning system. Diking and disconnected lithospheric plumbing are proposed to explain the Eocene breakup. After the first phase of continental breakup, two major phases of spreading influenced the Norwegian–Greenland Sea. Phase I (from C24 to C21r, �54 to 49 Ma) marks the earliest phase of spreading, probably initiated in the central and outer part of the Møre Basin. During this period, the formation of overlapping systems and pseudo-fault development, indirectly influenced by the proto-margin segmentation, suggests the presence of additional micro-plates in the Norwegian-Greenland Sea. We also observed a significant change in the oceanic spreading system in the late Early Eocene. Based on observations from the surrounding areas, this supports a major and distinct tectonic and magmatic event in the Norwegian-Greenland Sea at around C21r (49-47.9 Ma), the beginning of a second phase. During Phase II, from C21r-C12 or possibly younger (48-<32 Ma) of the Norway Basin development, spreading rates decreased, spreading direction changed leading to the formation of unexpected N-S oriented oceanic fracture zones. Phase II probably coincides with the climax of extension and possibly local spreading that is suspected in the southern part of the Jan Mayen micro-continent forming a complex area of oceanic, transitional and continental fragments before its complete dislocation from East Greenland in Latest Oligocene.
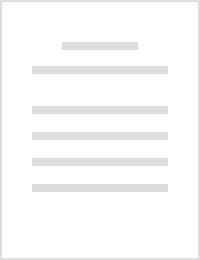
ABSTRACT Lithosphere extension and magmatism at volcanic passive margins Laurent GEOFFROY (1), La... more ABSTRACT Lithosphere extension and magmatism at volcanic passive margins Laurent GEOFFROY (1), Laurent GERNIGON (2), and Philippe WERNER (3) (1) Université de Bretagne Occidentale/IUEM, IUEM, UMR 6538, PLOUZANE, France ([email protected]), (2) Norges geologiske undersøkelse (NGU), P.O. Box 6315 Sluppen 7491 Trondheim, NORWAY, (3) Total Exploration, Paris La Défense, France We present onshore and offshore evidences suggesting that volcanic passive margins are distinct in origin and evolution from non-volcanic hyper-extended margins. Consecutively, they should not be integrated in a single evolutionary process and do not necessarily represent the ultimate stage of an hyper-extension with or without mantle exhumation. Volcanic passive margins usually form in mobile areas between cratonic areas which may have been submitted to long-term periods of divergence and convergence or strike-slip tectonics. In the NE-Atlantic, for example, a complete illustration of a Wilson cycle is illustrated between Greenland and Baltica cratonic areas. From the Devonian to the end of the Jurassic, the Caledonian orogenic crust has suffered from a number of wrench and extensional tectonic stretching episodes. The late-Jurassic/Early Cretaceous extension was severe, leading to extreme crustal thinning (e.g. Rockall Through, Vøring Basin, Lofoten Basin) and was followed by a longterm regional thermal subsidence of the NE-Atlantic lithosphere. Meanwhile, pre-thinning lithospheric thickness was restored progressively during �80 Myr, in spite of some tectonic reactivation occurring in Late Cretaceous (e.g. Outer Vøring Basin) resulting in little coeval stretching and thinning. During the Paleocene (or even earlier, especially in the Rockall area) a regional mantle melting event occurred. The mantle melted in specific locations but led ultimately to a large igneous province formation during the onset of breakup. The NE-Atlantic continental crust was at this time extremely heterogeneous due to its tectonic inheritance but we think that generally the lithosphere was much thicker than during the Jurassic-Cretaceous event, and thus much stronger. Although we must consider the existence of some extension during the latest Cretaceous and Paleocene, the main stretching and thinning event leading to volcanic passive margins formations and successful break-up occurred regionally during the Eocene, at the edge of the cratonic areas. In the NE-Atlantic, volcanic margins develop often along the thickest Phanerozoic crusts (such as Hatton Bank or Fugløy crusts, with cumulated thinning factor of solely �1.3 from the Palaeozoic). In some cases, they also appear to develop along much thinner crusts. However, in such cases a huge gap in time and dynamical contexts exists between the old Phanerozoic building and the final Latest-Cretaceous (?)-Eocene events that led to break-up. In such a situation, the initial extensional shaping of the crust should lead to a stronger rheology after lithospheric restoration. Therefore, the breakup might be difficult to reach without, notably, the providential input of magma within the lithosphere probably associated with localized thermal advection of the mantle lithosphere at craton edges. To conclude, any attempt to describe the breakup as a continuous and ultimate result of an extreme lithosphere thinning with mantle exhumation seems to us very hazardous when dealing with volcanic margins.
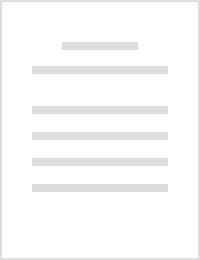
ABSTRACT The Møre margin is the result of a long period of rifting events influenced by different... more ABSTRACT The Møre margin is the result of a long period of rifting events influenced by different tectonic processes. The last extension phase of the Møre margin was accompanied by the onset of massive magmatic activity leading to the emplacement of seaward dipping reflector sequences (SDRS). However the previous events were probably characterized by a smaller amount of magmatism, like for a classic magma poor rifted margin. Several refraction studies across the Møre rifted margin have also revealed the occurrence of high velocity lower crustal bodies (LCB) under the distal SDRS wedge but also in more proximal positions of the margin (e.g. Kvarven et al., 2012). The nature, age and location of these LCBs are still questionable and represent key and primary parameters to understand the tectonic and crustal evolution of the volcanic rifted margin. Nature, age and geometry of these LCBs have major implications on the rheological and thermal evolution of the rift/margin system. In light of 2D potential field modelling combined with reflection and refraction seismic data, we reinvestigated the crustal nature of the Møre volcanic rifted margin and adjacent Jan Mayen corridor. In the proximal domain of the Møre volcanic rifted margin, our study shows that the LCBs most likely represent inherited crustal bodies and are not necessarily made of rift-related serpentinised mantle as previously proposed. In the distal margin, our preferred interpretation suggests that the outer LCBs are still made of relics of pre-rift lower continental crustal rocks, more or less intruded and/or underplated by Tertiary magmatic rocks. The seismic, magnetic and gravity data do not easily support large scale exhumation of serpentinised mantle in the inner and outer parts of the Møre Basin. Our model suggests that the Møre rift system evolved through a significant Late Jurassic-Cretaceous thinning phase. This rift episode led to a thin continental crust with pre rift lower crustal rocks but without perennial domains of exhumed and serpentinised mantle. This significant extensional event preceded a Late Cretaceous-Early Tertiary rift episode which is characterised by different and independent magmatic and lithospheric processes leading to breakup (e.g. diking and lithospheric plumbing).
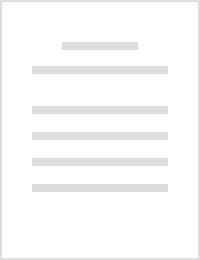
ABSTRACT Rifting processes exhibit deformation localization. In the dynamic model of rifting, ext... more ABSTRACT Rifting processes exhibit deformation localization. In the dynamic model of rifting, extensional deformation of the lithosphere cannot be localized without lateral rheological heterogeneity. In addition to a locally thickened crust, that has been adopted in previous dynamic modeling, magmatic underplating, commonly observed as high velocity lower crustal bodies along several volcanic rifted margins or magmatic rift, might have a significant effect on deformation localization or rift migration. Even though numerous prior studies have clearly showed that most of the rifted margins are affected by magmatism, few numerical studies have attempted to investigate the role and structural implication of magmatic underplating in rift and basin dynamics. In this study, a two-dimensional thermo-mechanical model is used to investigate the role of underplating in the localization of extension. We examine deformation localization possibly brought about by magmatic underplating in the dynamic response of lithosphere to an applied constant velocity at the boundary of the model. Melting processes are not invoked - an instantaneous underplating is simply assumed in our modeling. The first step of the investigation is to examine the effect of basic model parameters on the localization, in which we simply assume that underplated material is initially located at the base of the crust. Then, reactivated underplating during rifting is taken into account in order to investigate the origin of rift migration. The results from the numerical study show that magmatic underplating can bring about significant deformation localization and strongly influence the rift geometry. Rheological weakening produced by the underplating is dependent on the initial lithospheric structure, which is linked with the following two main physical effects: underplated material has (1) high temperature and (2) anomalous crustal rock composition. The former effect will disappear in a few million years after the underplating, and the latter one will be a principal factor in the later stage. Deformation localization is dependent on width, thickness and temperature of underplating, and our sensitivity study concludes that deformation will be more favorably localized for the model with narrower and thicker underplating with higher temperature. The relative importance of underplating to thickened crust depends on the lateral scale of heterogeneity; if the upward migration of melts is concentrated in a narrower region than the lateral scale of crustal heterogeneity, extensional deformation can be significantly localized more chiefly by the underplating. Lateral heterogeneity in the thickness of the underplating can lead to an asymmetric rift structure. Magmatic activity during the extensional process could result in the migration of rift. This migration depends on the timing and intensity of magmatic activity, as well as depending on the above mentioned basic parameters which describe the characteristics of the underplated material.
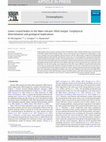
Tectonophysics, 2014
Understanding nature, structure and age of Lower Crustal Bodies (LCBs) and their relation to the ... more Understanding nature, structure and age of Lower Crustal Bodies (LCBs) and their relation to the crustal structure of the Møre margin (Mid-Norwegian margin), and in a more general way, of magma-rich rifted margins, is a key issue to decipher the tectono-magmatic processes found in volcanic rifted margins. In light of 2D potential field modelling combined with reflection and refraction seismic data, we reinvestigated the crustal nature of the Møre margin and adjacent Jan Mayen corridor. In the proximal domain, our study shows that the LCBs most likely represent inherited crustal bodies and not necessarily rift-related serpentinised mantle as previously proposed. To fit all geophysical observations, both lower and middle crustal layers need to be preserved over a large part of the Møre Basin. For the distal margin, the interpretation of the LCBs is more difficult. Our preferred interpretation is that they are mainly made of boudins of hyper-extended, pre-rift lower continental crustal rocks more or less intruded by Early Tertiary magmatic material. Our seismic, magnetic and gravity data does not easily support large scale exhumation of serpentinised mantle in the inner and is unlikely in the outer parts of the Møre Basin. The deep structures of the Mid-Norwegian magma rich rifted margin result from the poly-phase stretching and thinning of complex inherited crustal structures, locally intruded by Early Tertiary magmatic material.
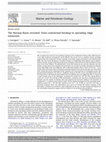
Marine and Petroleum Geology, 2012
We re-evaluate the structure and spreading evolution of the southeastern Norway Basin (NB) based ... more We re-evaluate the structure and spreading evolution of the southeastern Norway Basin (NB) based on a new high resolution aeromagnetic survey (NB-07). The survey covers a complete oceanic spreading segment from the continentaleoceanic transition of the Møre margin, off Norway to the aborted Aegir Ridge. The new survey documents a transform margin, an orthogonally rifted segment and an obliquesheared volcanic margin formed during the onset of breakup, observed from the East Jan Mayen Fracture Zone to the Faroe Platform. The detailed fabric of the NB revealed by the new data indicates that two distinct tectonic phases have reshaped the basin before the cessation of seafloor spreading and abortion of the Aegir Ridge in the Late Oligocene. After continental breakup, Phase I (from C24 to C21r, w52 to 49 Ma) marks the earliest phase of spreading, probably initiated in the central and outer part of the Møre Basin. During this period, competing oceanic segments led to the formation of overlapping systems and pseudo-fault development. We observe a significant change in the NB's oceanic spreading system in the late Early Eocene and, based on observations from surrounding areas, we suggest that this is a record of a major tectonic event in the NorwegianeGreenland Sea around C21r (49e47.9 Ma). During Phase II (from C21r to C10?, 48 to 28 Ma) of NB's development, spreading rates decreased, spreading direction changed, and the number of faulting with large displacement increased leading to the formation of unexpected NeS oriented oceanic fracture zones. The fan-shaped development of the spreading system initiated around C21r (w49e47.9 Ma) instead of C18eC17 (w40e38 Ma) or C24 (53.3e52.3 Ma) as previously proposed. These new observations were used to re-evaluate the tectonic evolution of the NorwegianeGreenland Sea and discuss some implications on the syn-and post-breakup development of the surrounding continental margins and the evolution of the Jan Mayen microcontinent.
Journal of the Geological Society, 2012
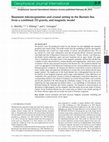
Geophysical Journal International, 2013
We present a new 3D geophysical model for the Barents Sea that highlights the basement properties... more We present a new 3D geophysical model for the Barents Sea that highlights the basement properties and crustal setting. The model results from the modelling of gravity and magnetic field anomalies and is based on a large number of seismic and petrophysical data. The set up consists of a water layer, sedimentary units that incorporate density variations associated with depth and time of deposition (Cretaceous-Cenozoic, Triassic-Jurassic, Late Palaeozoic and deeply buried sediments), upper and lower basement and an upper mantle. The upper crust is considered as the major source of the magnetic anomalies and has been divided into a number of units characterized by constant densities and magnetization, which show a good correlation with the main structural elements of the Barents Sea. The Southwest Barents Sea crust is an aggregation of allochthonous Caledonian terranes and autochthonous Archaean and Palaeoproterozoic complexes. We interpret the different crustal blocks in terms of distinctive lower, middle, upper and uppermost allochthonous terranes that can be linked with the major nappes onshore. The largest part of the North Barents Sea is distinguished from the rest of the shelf by its low-magnetic properties and its large crustal thickness. These differences are compatible with a geodynamic scenario in which an independent crustal block (Barentsia, not corresponding entirely to the island of Svalbard) was located between Baltica and Laurentia and became attached to the shelf during the Caledonian orogeny. To the east, the basement underlying the large mega-sag East Barents Basin, is an assemblage of Precambrian rocks deformed during the Timanian and Uralian orogenies. The basement is characterized by an alternation of high-magnetic and low-magnetic units that mimic the arcuate shape of Novaya Zemlya. In the Southeast Barents Sea, the crustal units are linked to the onshore geology of the Timan-Pechora region and are mostly the result of Timanian orogenesis.
Geological survey of Norway, …, 2009
Uploads
Papers by Laurent Gernigon